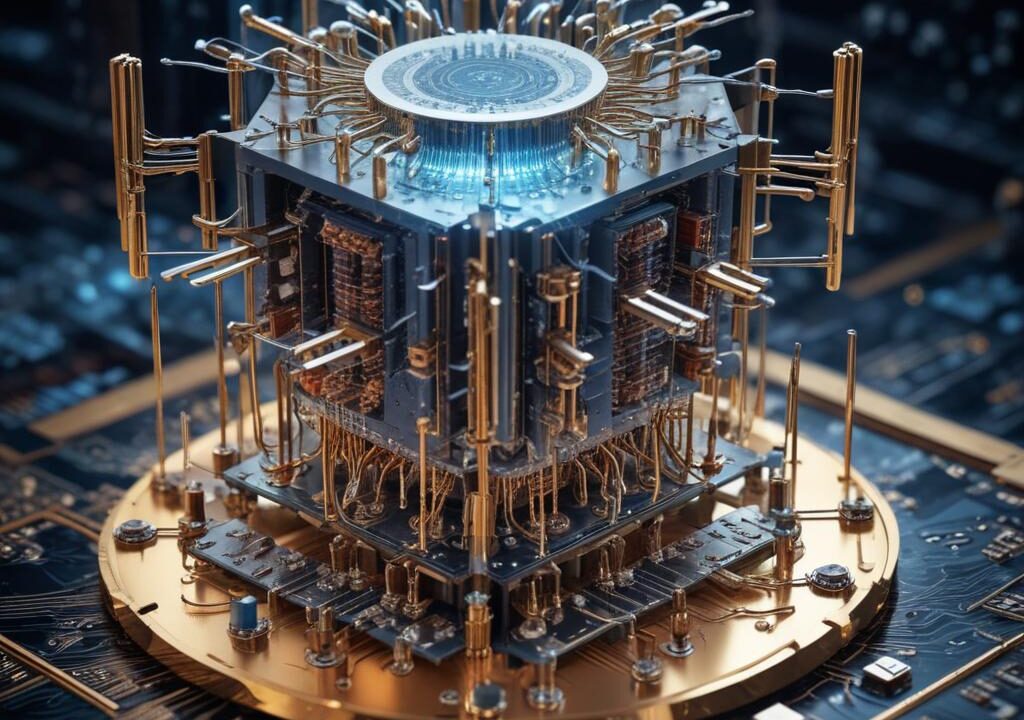
Unlocking the Future of Technology
Quantum computing represents a revolutionary leap in computing technology, poised to transform various industries and solve complex problems far beyond the reach of classical computers. Unlike classical computers that use bits as the basic unit of information, quantum computers leverage quantum bits or qubits. This fundamental shift enables quantum computers to process data in ways that classical computers cannot, leading to potential breakthroughs in cryptography, material science, medicine, and artificial intelligence.
The Basics of Quantum Computing
Qubits: The Quantum Bits
Classical computers use bits as the smallest unit of data, which can be either 0 or Quantum computers, on the other hand, use qubits. A qubit can exist in a state of 0, 1, or both simultaneously, thanks to the principle of superposition. This allows quantum computers to perform many calculations at once.
Superposition
Superposition is a fundamental principle of quantum mechanics that allows particles to be in multiple states simultaneously. In quantum computing, this means a qubit can represent both 0 and 1 at the same time. This property is harnessed to perform parallel computations, drastically increasing computational power.
Entanglement
Entanglement is another fundamental quantum principle where two or more qubits become interconnected such that the state of one qubit directly influences the state of another, regardless of the distance between them. This interconnection allows quantum computers to solve complex problems more efficiently than classical computers.
Quantum Gates and Circuits
Quantum gates manipulate qubits through operations similar to logic gates in classical computing. These gates form quantum circuits that execute quantum algorithms. Unlike classical gates, quantum gates can perform complex transformations on qubits, enabling powerful computational capabilities.
Quantum Algorithms
Quantum algorithms leverage the unique properties of quantum computing to solve problems more efficiently than classical algorithms. Some notable quantum algorithms include:
Shor’s Algorithm
Shor’s algorithm, developed by Peter Shor in 1994, revolutionized the field by demonstrating that quantum computers could factor large integers exponentially faster than classical computers. This algorithm poses a significant threat to classical cryptographic systems, which rely on the difficulty of factoring large numbers.
Grover’s Algorithm
Grover’s algorithm, created by Lov Grover in 1996, provides a quadratic speedup for unstructured search problems. While not as dramatic as the exponential speedup offered by Shor’s algorithm, Grover’s algorithm still significantly improves various search and optimization problems.
Quantum Fourier Transform (QFT)
The Quantum Fourier Transform is critical in many quantum algorithms, including Shor’s algorithm. QFT efficiently transforms quantum states and is crucial for solving problems related to periodicity and phase estimation.
Quantum Computing Models
Several models of quantum computing have been proposed, each with its approach to harnessing quantum mechanics for computation:
Gate-Based Quantum Computing
The gate-based model is the most widely studied and implemented form of quantum computing. It uses quantum gates to build circuits that perform specific algorithms. Companies like IBM, Google, and Rigetti Computing have developed gate-based quantum processors.
Quantum Annealing
Quantum annealing solves optimization problems by finding a system’s lowest energy state. D-Wave Systems is a pioneer in quantum annealing, with machines that solve specific optimization problems faster than classical computers.
Topological Quantum Computing
Topological quantum computing aims to use anyons, particles that follow different statistical rules than standard particles, to create robust qubits that are less prone to errors. While still theoretical, this approach promises greater fault tolerance and stability for quantum computations.
Quantum Hardware
Building quantum computers requires overcoming significant engineering challenges. Several technologies are being explored to create reliable qubits and scalable quantum systems:
Superconducting Qubits
Superconducting qubits are among the most advanced and widely used in current quantum processors. They use superconducting circuits cooled to near absolute zero to exhibit quantum behavior. Companies like IBM, Google, and Rigetti use this technology in their quantum processors.
Trapped Ions
Trapped ion qubits use ions confined in electromagnetic fields and are manipulated using lasers. This technology offers high-fidelity qubits and long coherence times, making it a promising candidate for scalable quantum computers. IonQ and Honeywell are leaders in trapped ion quantum computing.
Photonic Qubits
Photonic qubits use particles of light (photons) to encode and manipulate quantum information. This approach benefits from low-noise environments and easy integration with existing communication technologies. Companies like Xanadu and PsiQuantum are developing photonic quantum processors.
Topological Qubits
Topological qubits aim to use exotic particles called anyons to encode quantum information in a way that is inherently protected from errors. Microsoft is heavily invested in researching and developing topological qubits for future quantum computers.
Quantum Computing Applications
Quantum computing holds the potential to revolutionize numerous fields by solving problems that are currently intractable for classical computers:
Cryptography
Quantum computers pose both opportunities and threats to cryptography. Shor’s algorithm can break widely used cryptographic schemes like RSA, necessitating the development of quantum-resistant cryptographic algorithms. Simultaneously, quantum key distribution (QKD) offers unbreakable encryption based on principles of quantum mechanics.
Drug Discovery and Material Science
Quantum computers can simulate molecular structures and chemical reactions with unprecedented accuracy. This capability can accelerate drug discovery, leading to new disease treatments and enabling the design of advanced materials with tailored properties.
Optimization Problems
Many real-world problems, such as supply chain logistics, financial portfolio optimization, and traffic management, can be formulated as optimization problems. Quantum annealers and gate-based quantum computers can solve these problems more efficiently than classical computers.
Machine Learning and AI
Quantum computing has the potential to enhance machine learning and artificial intelligence by accelerating training times for complex models and improving the efficiency of data processing. Quantum algorithms like quantum support vector machines and quantum neural networks are being developed to leverage this potential.
Climate Modeling
Quantum computers can more accurately model complex systems, such as climate patterns, than classical computers. This capability can improve our understanding of climate change and help develop more effective strategies for mitigation and adaptation.
Challenges and Future Directions
While quantum computing holds immense promise, several challenges must be addressed to realize its full potential:
Error Correction and Decoherence
Quantum systems are highly susceptible to errors due to decoherence and external noise. Developing robust error correction methods and fault-tolerant qubits is critical for building reliable quantum computers.
Scalability
Scaling quantum processors to many qubits while maintaining coherence and low error rates is a significant engineering challenge. Innovations in hardware design, fabrication, and system architecture are needed to achieve scalable quantum computing.
Software and Algorithms
Developing efficient quantum algorithms and software tools is essential for effectively leveraging quantum hardware. This includes creating high-level programming languages, compilers, and optimization tools tailored for quantum systems.
Workforce Development
Building a skilled workforce with expertise in quantum computing, quantum mechanics, and related fields is vital for advancing the technology. Educational programs, research initiatives, and industry collaborations are crucial for training the next generation of quantum scientists and engineers.
Conclusion
Quantum computing represents a paradigm shift in processing information, offering unprecedented computational power for solving complex problems. With ongoing advancements in hardware, software, and algorithms, quantum computing is poised to revolutionize various industries, from cryptography and drug discovery to optimization and artificial intelligence. While significant challenges remain, the potential benefits of quantum computing make it a fascinating and promising field of research and development. As we continue to unlock the mysteries of quantum mechanics, the future of quantum computing holds limitless possibilities for transforming our world
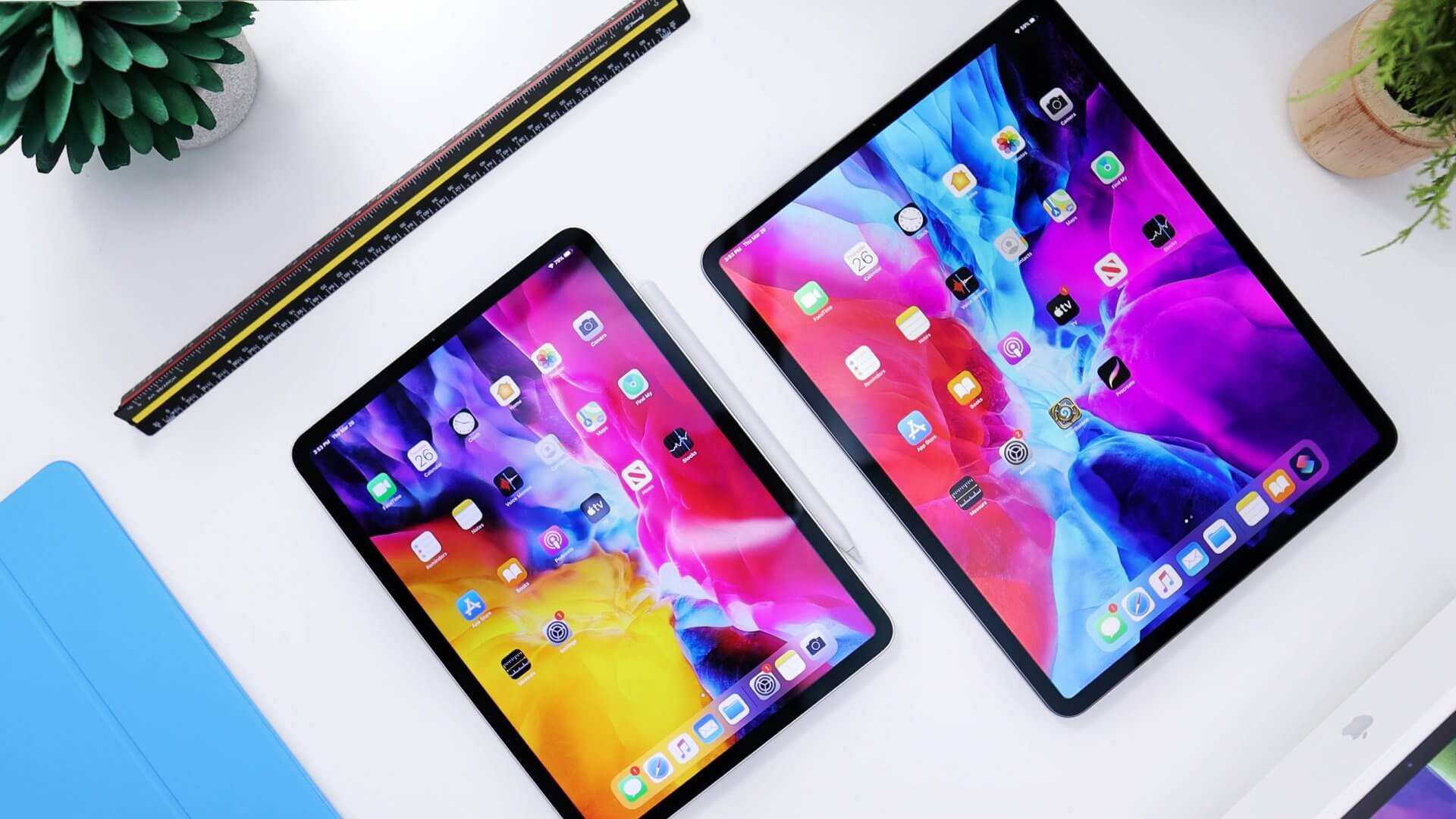
Review Title
There are many variations of passages of Lorem Ipsum available, but the majority have suffered alteration in some form, by injected humour, or randomised words which don't look even slightly believable. If you are going to use a passage of Lorem Ipsum, you need to be sure there isn't anything embarrassing hidden in the middle of text.If you use this site regularly and would like to help keep the site on the Internet, please consider donating a small sum to help pay for the hosting and bandwidth bill. There is no minimum donation, any sum is appreciated - click here to donate using PayPal. Thank you for your support.
Pros
- Pors Item One
- Pors Item Two
- Pors Item Three
- Pors Item Four
- Pors Item Five
Cons
- Cons Item One
- Cons Item Two
- Cons Item Three
- Cons Item Four
- Cons Item Five